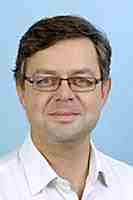
Dr. Matthias Troyer is a professor of Computational Physics at ETH Zürich. Before that, he finished University Studies in “Technischer Physik” at the Johannes Kepler Universität Linz, Austria, as well as Diploma in Physics and Interdisciplinary PhD thesis at the ETH Zürich.
His research interest and experience focuses on High Performance Scientific Simulations on architectures, quantum lattice models and relativistic and quantum systems. Troyer is known for leading the research team of the D-Wave One Computer System. He was awarded an Assistant Professorship by the Swiss National Science Foundation.
Luke Muehlhauser: Your tests of D-Wave’s (debated) quantum computer have gotten much attention recently. Our readers can get up to speed on that story via your arxiv preprint, its coverage at Scott Aaronson’s blog, and Will Bourne’s article for Inc. For now, though, I’d like to ask you about some other things.
If you’ll indulge me, I’ll ask you to put on a technological forecasting hat for a bit, and respond to a question I also asked Ronald de Wolf: “What is your subjective probability that we’ll have a 500-qubit quantum computer, which is uncontroversially a quantum computer, within the next 20 years? And, how do you reason about a question like that?”
Matthias Troyer: In order to have an uncontroversial quantum computer as you describe it we will need to take three steps. First we need to have at least ONE qubit that is long term stable. The next step is to couple two such qubits, and the final step is to scale to more qubits.
The hardest step is the first one, obtaining a single long-term stable qubit. Given intrinsic decoherence mechanisms that cannot be avoided in any real device, such a qubit will have to built from many (hundreds to thousands) of physical qubits. These physical qubits will each have a finite coherence time, but they will be coupled in such a way (using error correcting codes) as to jointly generate one long term stable “logical” qubit. These error correction codes require the physical qubits to be better than a certain threshold quality. Recently qubits started to approach these thresholds, and I am thus confident that within the next 5-10 years one will be able to couple them to form a long-time stable logical qubit.
Coupling two qubits is something that will happen on the same time scale. The remaining challenge will thus be to scale to your target size of e.g. 500 qubits. This may be a big engineering challenge but I do not see any fundamental stumbling block given that enough resources are invested. I am confident that this can be achieved is less than ten years once we have a single logical qubit. Overall I am thus very confident that a 500-qubit quantum computer will exist in 20 years.
Luke: At the present moment, which groups seem most likely to play a significant role in the final development of an early large-scale (uncontroversial) quantum computer?
Matthias: There is quite a large number of groups that may be involved. The technologies that I see as most promising regarding scalability and quality of qubits are superconducting qubits, topological qubits and ion traps.
Luke: Now back to your co-authored paper “Defining and detecting quantum speedup,” now published in Science. You an your coauthors “found no evidence of quantum speedup [in the D-Wave Two machine] when the entire data set is considered and obtained inconclusive results when comparing subsets of instances on an instance-by-instance basis.”
Do people who have investigated a D-Wave machine at some length tend to think that D-Wave has a “true” quantum computer but hasn’t been able to conclusively show it yet, or do they tend to think D-Wave doesn’t yet have a true quantum computer?
Matthias: The answer to this controversial question depends on the definition of the words “quantum” and “computer”. Let’s first talk about “computer”. The D-Wave devices are special purpose devices, built for one particular purpose, namely the solution of discrete optimization problems. Since they solve a computational problem they may be called “computers”, but in contrast to your standard personal computers, which can perform many different tasks they are not “universal computers” but special purpose computers. Nowadays many people automatically assume that a “universal computer” is meant when the term computer is mentioned, and one should thus explicitly state that the D-Wave devices are not universal computers but special purpose ones.
D-Wave thus cannot be, and nobody has ever claimed so, to be a universal quantum computer. While nobody would argue against the D-Wave device being called a computer, the question of it being “quantum” is more controversial. In a previous paper in Nature Physics we have presented evidence that the behavior of the D-Wave devices is consistent with that of a “quantum annealer” working at nonzero temperature, and another recent paper has shown that entanglement is present in the devices. The devices hence use quantum effects for computing. However, another paper has shown that the behavior of a quantum annealer for the problems on which we tested the device can also be described by a purely classical model. Some people thus argue that while the device may use quantum effects it might in the end be a classical device, just like the CPU in your PC, where the transistors also uses quantum effects at some level.
The important question thus is whether the device can have quantum speedup, which means that it outperforms any classical device by a larger and larger ratio as the size of problems is increased. If that should be shown for some class of problems then nobody would argue about the quantum nature of the device. On the other hand, as long as its computational powers are never more than that of a classical computer, one can argue that from a computational point of view it is effectively a classical device even if it uses quantum effects to arrive at the answer.
To answer your question more concisely. If by a “true quantum computer” you mean a universal quantum computer, then D-Wave is not a true quantum computer and nobody has ever claimed that it is one. If you are content with it being a special purpose quantum computer, i.e. a a “true quantum annealer”, then this question depends on what you mean by “true”: it’s behavior seems to be consistent with what we expect from a quantum annealer, but since we have so far not seen evidence of quantum speedup, i.e. that quantum effects help it outperform classical computers, one can argue that it may effectively be a classical device.
Luke Muehlhauser: Do you have an opinion about the impact that large-scale quantum computers (universal or not) are likely to have on AI and machine learning methods? E.g. Aimeur et al. (2013) seems optimistic about speeding up some machine learning methods using variants of Grover’s algorithm.
Matthias Troyer: I haven’t read that specific paper but my sense is that quantum machine learning will be most useful for learning about quantum data that comes out of quantum experiments. Applying quantum algorithms to classical data, even quantum algorithms need to read the data first, and thus cannot do better than linear effort in the size of the data. With efficient polynomial time algorithms existing for many machine learning problems one will have to have better ideas than just speeding up classical machine learning algorithms on quantum hardware.
Luke Muehlhauser: Thanks, Matthias.
Did you like this post? You may enjoy our other Conversations posts, including: